Investigation of Air Entrainment and Turbulent Structures in Breaking Surge Waves
Project Snapshot
- Project Title: Investigation of Air Entrainment and Turbulent Structures in Breaking Surge Waves
- Type: Internship - Mitacs globalink research internship
- Project Report: View Article (PDF)
- Concepts:
- Volume of Fluid (VOF) Method
- Turbulence Modeling
- Method of Characteristics (MOC)
- Surge Wave Dynamics
- Quadrant Analysis
- Large Eddy Simulation (LES)
- Duration: 2022
- Skills Developed:
- CFD Software: OpenFOAM
- CAD Software: SolidWorks
- Data Analysis: pandas, numpy
- Post-Processing Tools: ParaView, matplotlib, MATLAB
- Others: Compute Canada
Project Overview
1. Objective
To investigate the air entrainment patterns and their connection to the turbulent structures of breaking surge waves with Froude numbers higher than 1.6, using Large Eddy Simulation (LES) and Volume of Fluid (VOF) methods.
2. Key Contributions
- Advanced Simulation Techniques: Employed a combination of VOF and LES to simultaneously capture air entrainment and turbulent structures in breaking surge waves.
- Turbulent Kinetic Energy Analysis: Explored the linkage between turbulent kinetic energy distribution and air entrainment patterns.
- Quadrant Analysis and Q-Criterion Application: Examined the role of spanwise perturbations in the development of turbulent structures using quadrant analysis and the Q-criterion.
Introduction and Background
1. Breaking Surge Waves and Turbulence
- Surge waves are transient open channel flows resulting from sudden changes in flow depth or velocity, occurring in both man-made hydraulic structures and natural systems.
- Breaking surge waves occur at Froude numbers higher than 1.6 and exhibit complex dynamics due to turbulence and air entrainment interactions.
- Turbulence in breaking surge waves is induced by the velocity gradient across the surge and the phase discontinuity at the front, leading to the formation of a shear layer originating at the surge toe.
2. Research Motivation
- Understanding the turbulent structures and air entrainment in breaking surge waves is essential for predicting flow behavior in hydraulic engineering applications.
- Previous studies have not fully captured the three-dimensional turbulent structures and their connection to air entrainment patterns in breaking surge waves.
- This study aims to fill this gap by utilizing advanced simulation techniques to investigate these phenomena.
Methodology
1. Simulation Framework
- Software Utilized: Employed OpenFOAM v7, an open-source computational fluid dynamics platform.
- Solver Selection: Used the InterFoam solver, which employs the Volume of Fluid (VOF) method for simulating incompressible, multiphase free-surface flows.
- Turbulence Modeling: Implemented Large Eddy Simulation (LES) to capture larger-scale turbulent motions, with subgrid-scale viscosity modeling the impact of smaller-scale motions.
- VOF Method: Used the Van Leer Total Variation Diminishing (TVD) scheme for accurate phase separation and interface capturing between water and air.
- Pressure-Velocity Coupling: Employed the PIMPLE algorithm for pressure–velocity coupling in the Navier–Stokes equations.
2. Simulation Setup
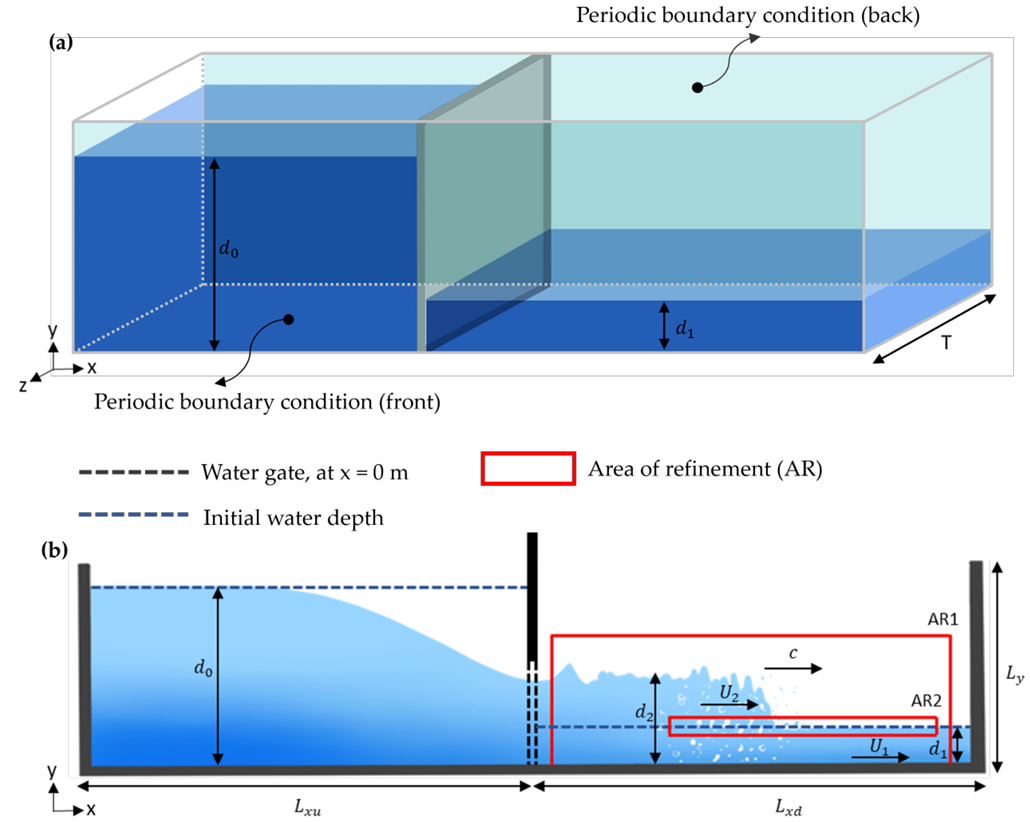
Fig1. Boundary conditions and grid refinement
- Computational Domain: Designed to accommodate 8 seconds of simulation before boundary effects influenced the results.
- Data Collection: Analysis focused on the period between 4 and 6 seconds when the turbulence at the wave front was fully developed.
- Boundary Conditions: Implemented spanwise periodic boundary conditions to simulate the three-dimensional nature of vortical structures.
- Grid Refinement: Applied two areas of refinement to resolve the turbulent structures accurately, particularly around the surge toe where the shear layer forms.
Results and Analysis
1. Turbulent Kinetic Energy Distribution
- Observed higher values of resolved TKE around the surge front in both air and water, with peaks near the surge toe for both Froude numbers.
- Subgrid-scale TKE exhibited higher values around the surge toe, indicating significant small-scale turbulence in this region.
- The ratio of resolved to total TKE remained above the recommended value for LES, ensuring accurate simulation of turbulence.
2. Water Depth Perturbations
- Instantaneous water surface profiles showed larger perturbations immediately behind the surge toe.
- Normalized water surface perturbations peaked at higher values for higher Froude numbers, consistent with experimental observations.
- Skewness analysis indicated extreme positive water surface perturbations near the toe, attributed to the steep wave front and high TKE levels.
3. Air Entrainment Patterns
- Air concentration profiles were extracted at multiple locations between the surge toe and heel.
- Instantaneous profiles often peaked at the depth of the shear layer, demonstrating the influence of TKE on air entrainment.
- Averaged profiles showed consistent patterns with laboratory observations, validating the simulation approach.
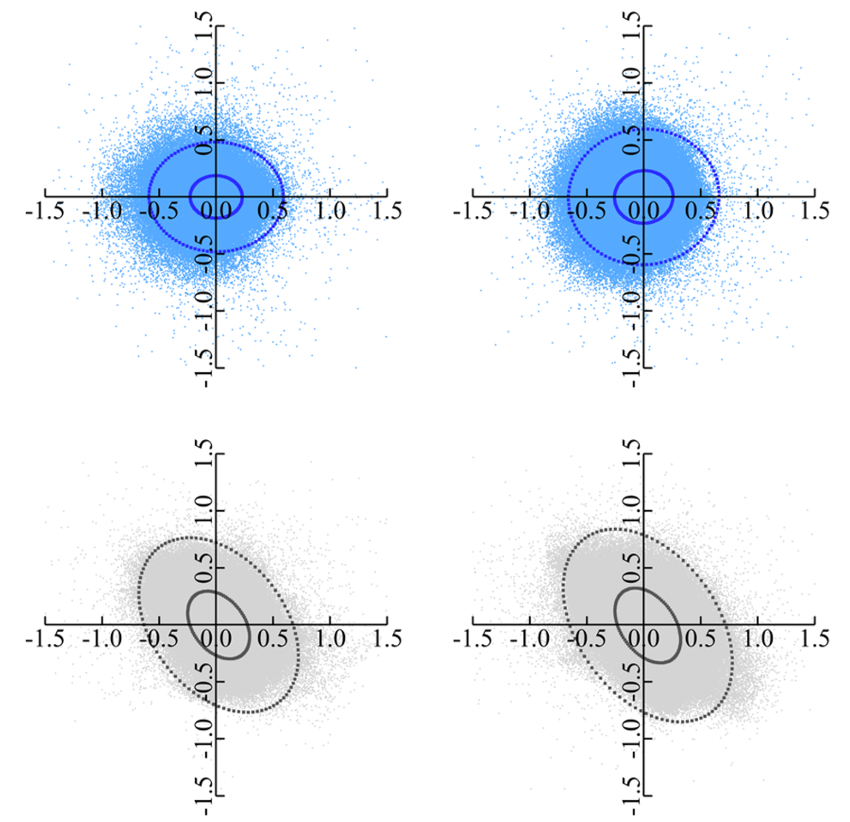
Fig2. Quadrant analysis
4. Velocity Perturbations and Quadrant Analysis
- Analyzed instantaneous velocity perturbations in different planes to quantify turbulent structures behind the breaking surge waves.
- Standard Deviational Ellipses (SDEs) were used to characterize the spatial distribution of perturbations.
- Quadrant analysis indicated that perturbations were oriented towards the second and fourth quadrants, leading to positive TKE production.
- The Q-criterion was employed to visualize coherent structures, highlighting the three-dimensional nature of turbulence in breaking surge waves.
5. Vortex Structure and Quadrant Analysis in Breaking Surge Waves
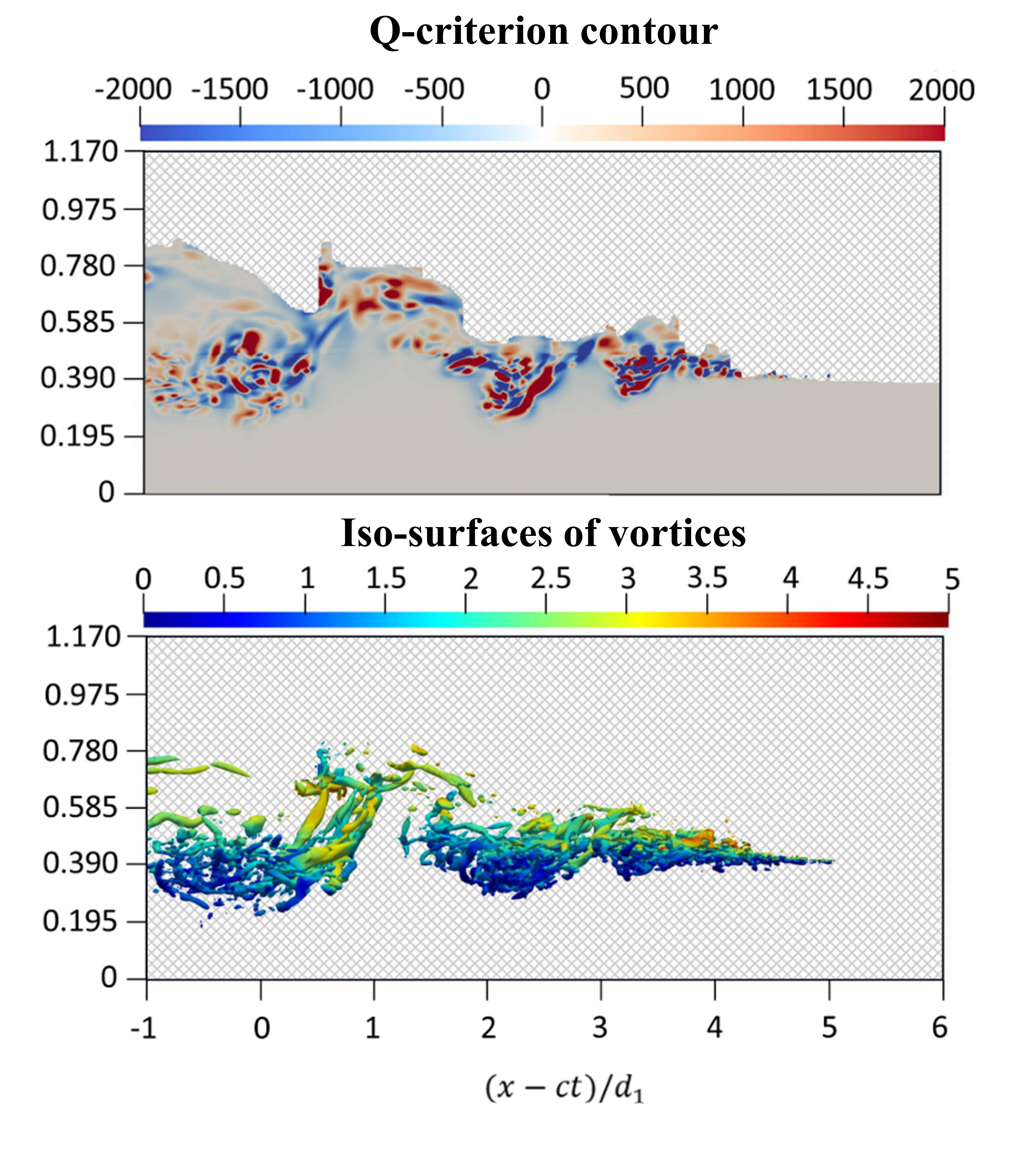
Q-criterion and isosurfaces
- Q-Criterion Visualization: The Q-criterion was employed to visualize the formation, rolling, and merging of coherent structures. These plots consistently revealed vortex accumulation near the toe and behind the breaking surge front.
- Instability Mechanisms: The vortices were generated by two primary instability mechanisms and advected behind the surge.
- The shear instability was oriented in the xy-plane, characterized by the gradient of the x-component of velocity in the y-direction. This resulted in 2D vortex structures near the toe.
- The depth discontinuity instability also occurred in the xy-plane, caused by the phase or depth gradient across the surge front in the x-component.
- Velocity Perturbations in z-Direction: The velocity perturbation component in the z-direction (w) was critical for fully cascading turbulent flow. It was comparable in magnitude to the velocity perturbation component in the y-direction (v), indicating a fully developed three-dimensional turbulent flow.
Conclusions
Summary of Findings:
- The study successfully investigated the turbulent structures across breaking surge waves with Froude numbers of 1.71 and 2.13 using a three-dimensional LES model.
- By employing the VOF method, the simulations accounted for air entrainment and linked it with coherent structures across the surge wave.
- Identified two mechanisms contributing to instability: shear layer formation due to velocity gradient at the toe, and wave breaking at the air–water interface due to phase discontinuity.
- Water surface perturbation analysis revealed extreme perturbations near the toe, attributed to the steep wave front and high TKE levels.
- Simulated air concentration profiles were consistent with existing laboratory observations, validating the modeling approach.
- Spanwise perturbations played a significant role in the development of fully developed turbulent structures, contributing to the three-dimensional nature of the flow.
Implications and Future Work:
- The findings enhance the understanding of turbulence and air entrainment in breaking surge waves, which is essential for hydraulic engineering applications.
- Future work should focus on studying isotropic behaviors near the surge front, behind the surge, and in the toe region to further elucidate the three-dimensional turbulent structures.
Final Remarks
This study provides valuable insights into the complex dynamics of breaking surge waves, particularly the turbulent structures and air entrainment patterns. The use of advanced simulation techniques, including LES and VOF methods, allowed for a detailed examination of the flow phenomena, contributing to the broader understanding of transient open channel flows in hydraulic engineering.